Welcome to The Gameful Scientist! This newsletter explores the intersection of scientific discovery and creativity. Enjoy!
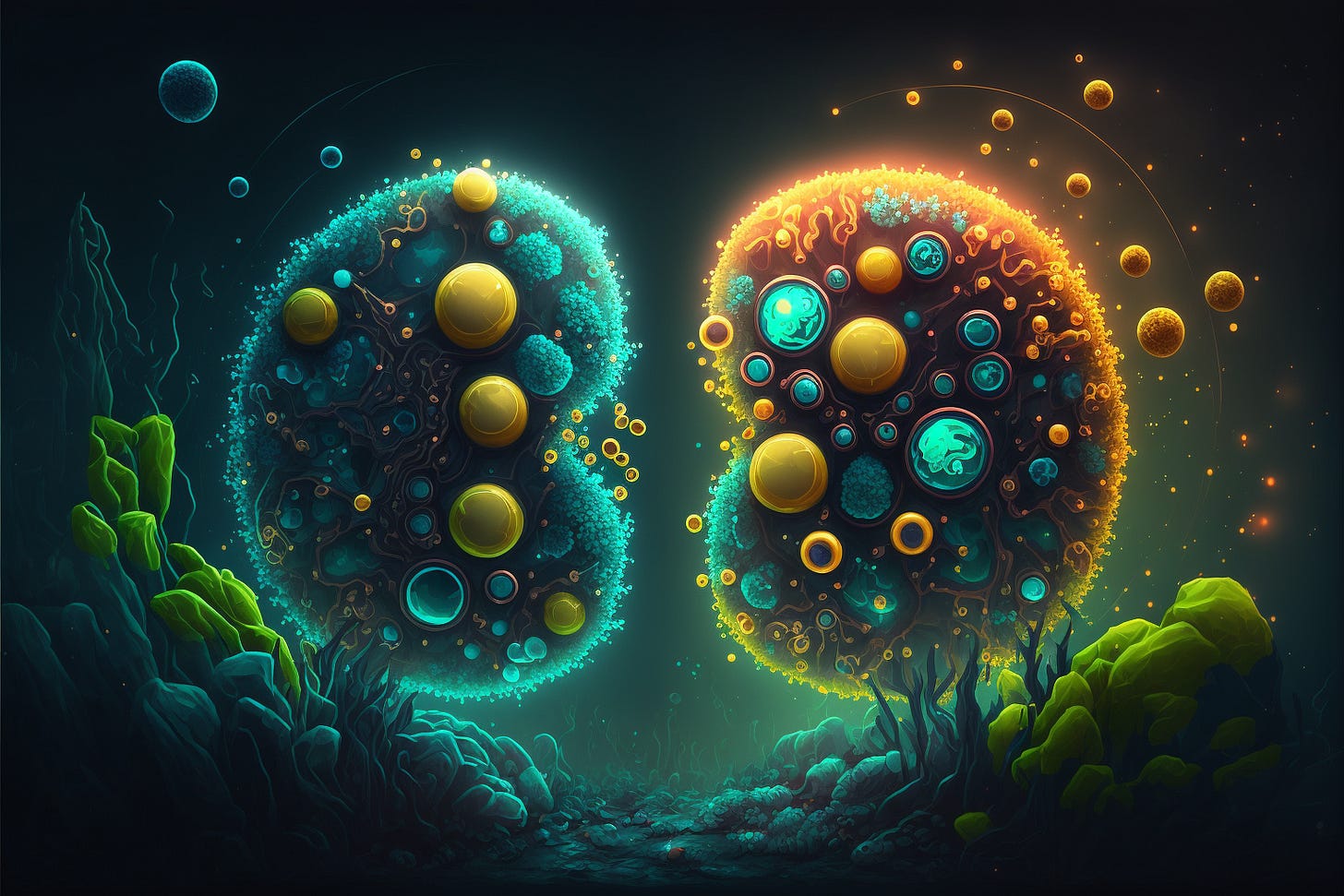
Imagine controlling living cells like characters in a video game. That’s the idea behind biotic games, where we’re reaching a new level of interaction between the human and biotic worlds.
In this article, we’ll explore the exciting genre of biotic games and the intersection of human-biology interaction (HBI) and the gaming industry. By treating microbes as “living computers”, we'll break down the components that make up biotic games, including bioware, hardware, and user interfaces. We'll highlight Pac-Euglena, a biotic game that serves as a prime example of the potential of this emerging field, and speculate on the future of this genre.
Welcome to Level 4.
The Evolution of Gaming
From pixelated aliens to talk of the Metaverse, the evolution of video games is an interesting story. Its roots can be traced back to a science fair in the 1940s, where Dr. Edward Uhler Condon introduced the first gaming machine based on the ancient game of Nim. The commercial gaming industry was born in 1967 with the launch of the Brown Box, the first digital gaming console. But, it was the arrival of arcade games in the 1970s, such as Atari's Pong, that sparked a revolution in gaming and marked the beginning of competitive play, where players would record their high scores and try to make their way to top of leaderboards.
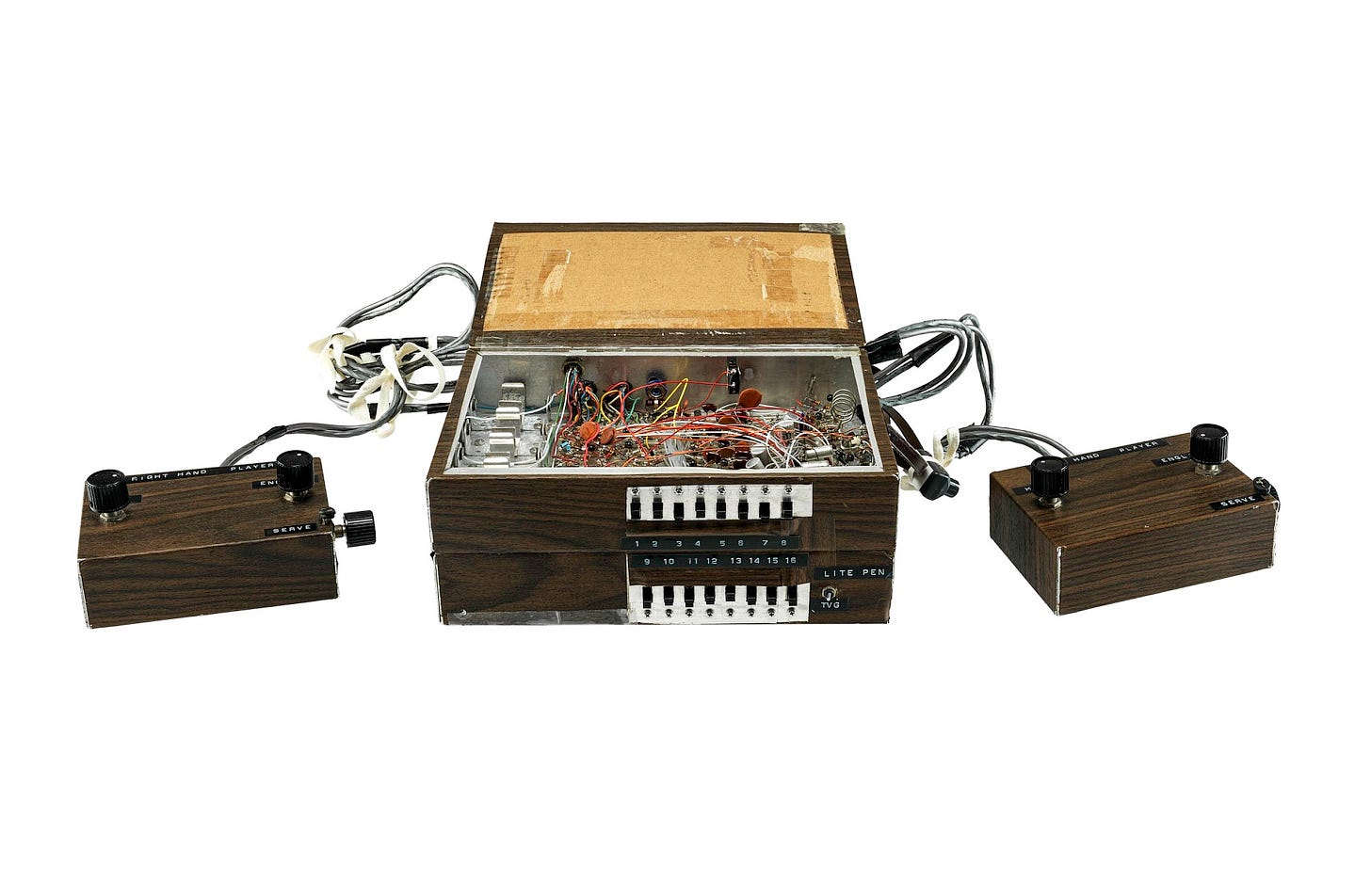
The rise of personal computers and the internet in the 1980s and 1990s made multiplayer gaming possible, and online gaming changed the industry forever. The growth of mobile gaming, driven by the iPhone, elevated the experience to even greater heights.
Now, we stand at the brink of a new era in gaming, driven by virtual reality (VR), augmented reality (AR), and mixed reality (MR). This phase of gaming promises to blur the lines between reality and fantasy, with VR headsets like Oculus allowing players to "live" inside a 3D world and AI bringing non-playable characters to life. Biotic games, by incorporating real biological materials and processes into the gaming world, represent a new frontier in the evolution of gaming.
Human-Biology Interaction
HBI is a new interdisciplinary field that explores the relationship between humans and living systems at the microscopic level. It's similar to Human-Computer Interaction (HCI), but with a focus on using living systems, like cells.
MIT’s Living Bits highlights some pretty incredible projects in the field of HBI.
In science-based games, HBI provides a unique gaming experience. Discovery games like Foldit and Eterna allow players to manipulate DNA and RNA virtually, but they are primarily simulations. While the best player-generated designs can be tested in a lab, players don't directly interact with biology. This sets HBI apart and opens up new opportunities for gaming and learning about the microscopic world. HBI presents a fresh way to study and understand living systems, potentially yielding new insights and practical uses.
Tiny Living Computers
Our bodies are home to trillions of microbes, which can be thought of as tiny living computers. They are simple organisms that use only a few processes and genetic parts, making them great for biological computing. Microbes are often compared to computers because they can receive inputs, process them, and create outputs.
In synthetic biology, or synbio, scientists manipulate biological processes by breaking them down into genetic parts and putting them back together in a new way. Like our own cells, microbes use DNA to store information, but instead of using the binary code (0 or 1) like computers, they use a four-letter code (A, C, T, G). Engineers use programming languages and software to design genetic circuits with these letters, similar to how they would design electrical circuits. These circuits help the microbes to sense, compute, and respond to different things by turning the DNA into useful proteins.
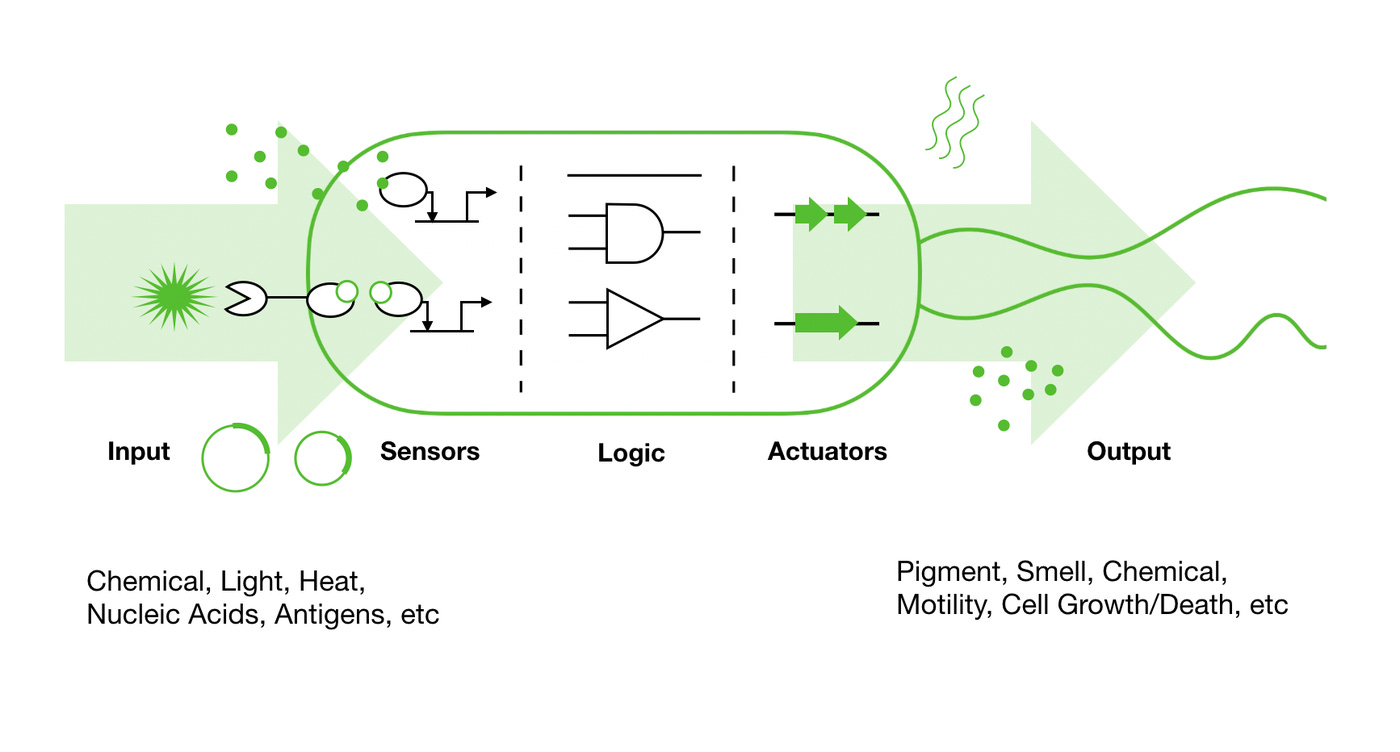
Synbio is poised to become a major player in the trillion dollar bio-economy and has many practical applications, such as producing cheaper drugs and more accurate bio-sensors. It also holds promise for more playful ambitions.
BioArt, for instance, blends science and art by creating unique, living pieces of art using genetically modified bacteria. These are referred to as bacterial paintings. Unlike traditional paintings that stay unchanged after the brushstroke, bacterial paintings are alive and can evolve based on temperature, time, and other external factors. The final product is a combination of the artist's design, incubation conditions, and the living cells themselves. This ability to program cells offers a range of possibilities, including a new genre in gaming.
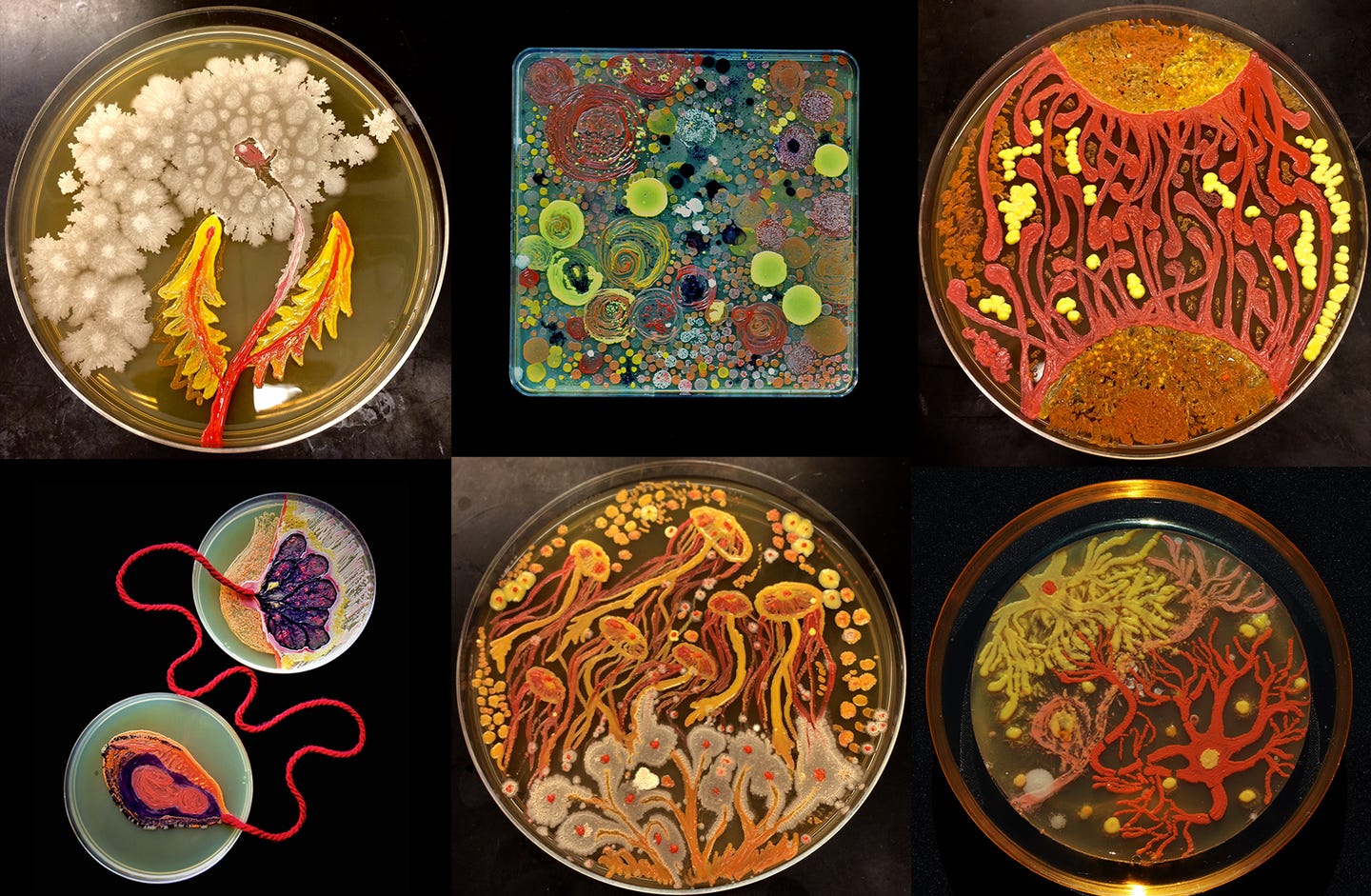
The Building Blocks of Biotic Games
Biotic games can be broken down into three main components: bioware, hardware, and user interfaces (UI).
Bioware refers to the living organisms, like microbes, that are used in these types of games. Genetic parts are used to program these organisms to respond to stimuli like light or chemicals, customizing the gaming experience.
Hardware, such as a biotic processing unit (BPU), serves as the bridge between the bioware and the player. The BPU holds the microbes and helps the player observe and control their reactions to the game. BPUs can be made using different methods, such as small, cheap electronics like those found in an Arduino, or with special equipment for viewing the microbes like webcam microscopes. The BPU helps measure what's happening with the living organisms, helps keep the game going smoothly, and allows for multiple games to be played at once.
UI enable human interaction with biotic games. This can be through touch screens, joysticks, or any other device that facilitates human-microbe interaction. UI can also set the rules of the game or allow for open play.
Case Study: Pac-Euglena
Several biotic games have emerged over the years, but we'll focus on Pac-Euglena, developed by the Riedel-Kruse Lab, a pioneer in the field of biotic games.
Pac-Euglena uses a microbe called Euglena as the main character. Euglena is special because it has a tail-like structure called a flagellum that helps it move and it moves towards light, a behavior known as phototaxis.
The Euglena are housed in a small container called a microfluidic chip. This chip is designed to resemble the classic video game Pac-Man and acts as the BPU, connecting the living microbe to the player. Players use a keyboard or joystick to turn on LED lights in the chip and direct the movement of the Euglena by creating a light gradient. The goal is to navigate the Euglena through the maze and collect virtual Pac-Dots to earn points, similar to the original Pac-Man game.
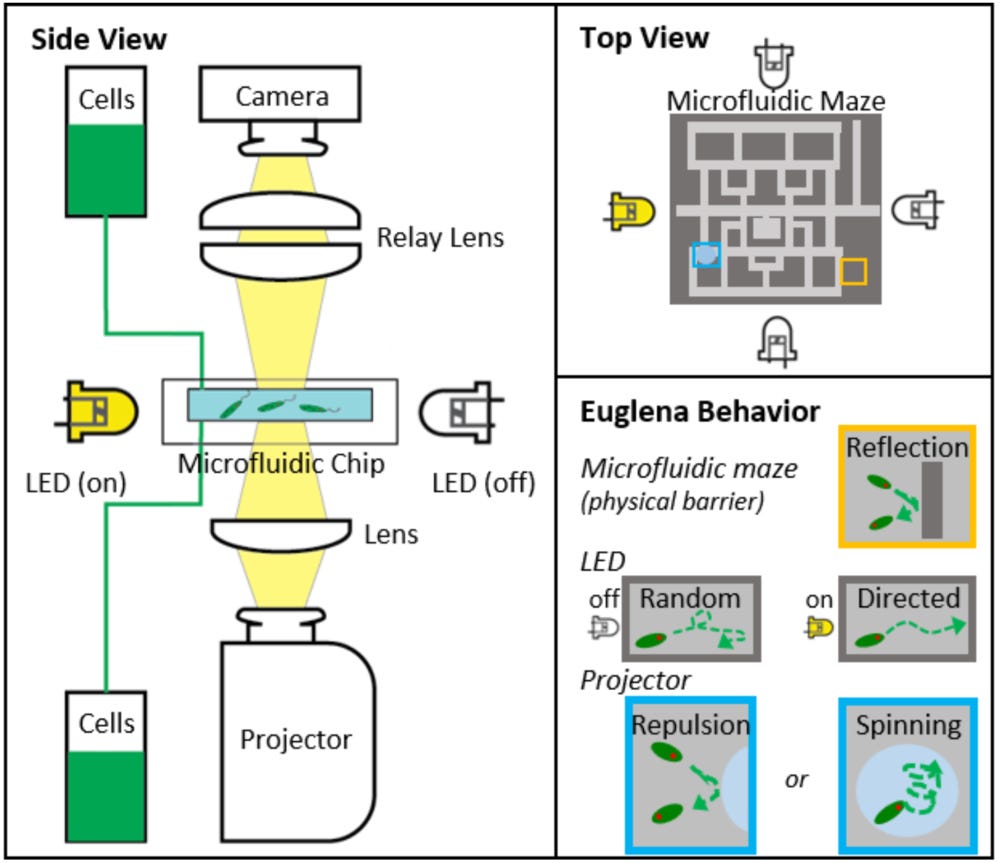
Computer vision is used to track the movement and interactions of each Euglena with the virtual overlay. At the start of the game, one random Euglena is designated as the Pac-Man character and the player earns points by guiding it to collect Pac-Dots. A virtual "ghost" is also introduced for extra challenge. If the Euglena comes into contact with the ghost, a bright blue light is projected from below the chip, causing the cell to spin in place for 5 seconds. This showcases the game's ability to use physical stimuli to control and interact with the living microbe.
Here’s an overview of the iterative game design process for Pac-Euglena.
Design Consideration for Biotic Games
Designing biotic games presents unique challenges compared to traditional video games.
While we just spent a section comparing microbes to computers, it’s an imperfect analogy. Biology is inherently messy and random, making it both a blessing and a curse for game design. On one hand, this can be used to create a sense of realness in playing with living systems, like a natural set of dice. On the other hand, game designers must find a balance between controlling variations for smooth gameplay and incorporating them for unpredictability.
Here are several design rules to consider when creating a biotic game:
Choose the right bioware and BPU: The bioware should be small and easily housed, such as microbes or DNA/RNA, so that the game can be low cost and require minimal intervention.
Match the scales of the human player and the microbe: The speed of biology in biotic games cannot be changed, but the difficulty can be adjusted by using magnification and screen size. For example, magnifying small microbes as large objects on a small screen creates fast-paced and unpredictable gameplay, while magnifying them as small characters on a larger screen makes them slower and easier to follow.
Control biological variability with hardware and software solutions: Analog joysticks can be used to adjust the intensity of light stimulus, reducing cell response variability over time. Adding virtual objects to the game can also provide narrative and adjust the dynamics.
Convey the realism of biology to players: The underlying biology and biotechnology should be transparent to the player in order to convey the "realness" of HBI and differentiate the biotic game from a simulation.
The Future of Biotic Games
Biotic games are full of possibilities and potential. As the tech advances, the chances of these types of games becoming as popular as traditional video games grows. Although playing with living cells might may prevent it from reaching quite that level, they have the potential to become a major genre due to the unique and unparalleled gaming experience they offer.
A key factor in the success of biotic games is the development of a general-purpose BPU. A BPU, standardized for use across different games, would make it easier for game developers to create and distribute biotic games, like how traditional video games are played on a gaming console. The BPU would be able to house different types of bioware, and integrate with future UI tech. Widespread adoption of BPUs for different types of games could result in a shift towards incorporating more living systems in game design and development.
Specialized, standardized, and interchangeable genetic parts are another important factor in the success of biotic games.
Dr. Drew Endy, one of the pioneers of standardizing these parts, says:
“We tend to think of biology as something that happens to us. But more and more, we are happening to biology. We’re in an era, scientifically, where we can express our intentions into the very kernel of life to allow for possibilities that are simply never going to exist otherwise.
Microbes will be designed for specific games, and all game functions will be physically implemented at the microscopic scale, without the need for digital overlays, like in Pac-Euglena. Cell may even be engineered to self-arrange and produce text on a screen that relays game instructions to the player.
We can also take some lessons from evolutionary “game theory”. This is a branch of math and biology that studies the evolution of social and economic behavior in cells. It’s based on the idea that cells interact with each other and compete for resources in their environment. In biotic games, players can program cells with genetic traits, such as speed, size, or survival abilities, for use in competitive play. Players can use their designed cells to compete for resources, similar to the game Factorio, where effective resource management and strategic decision-making are key to success.
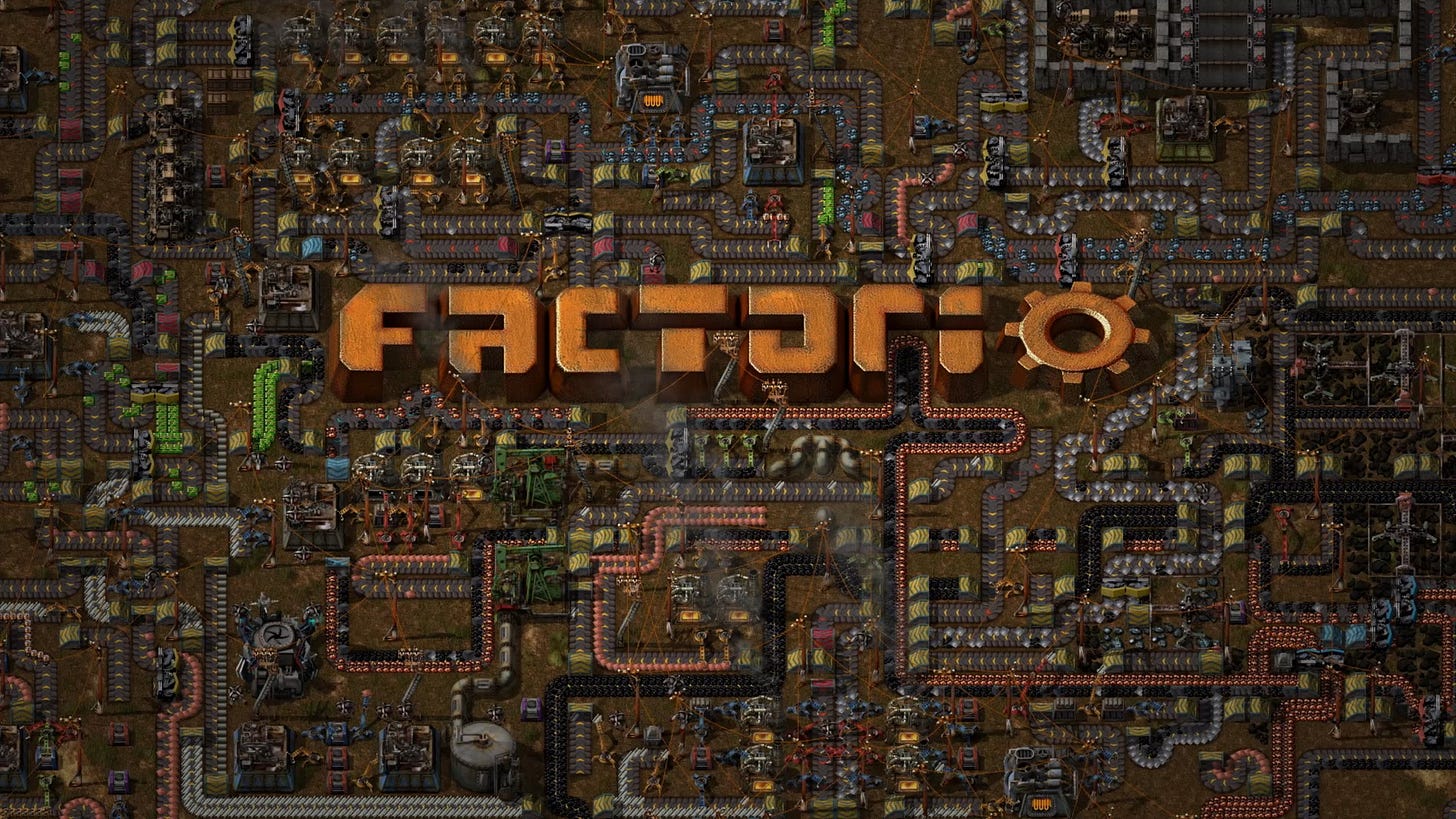
Finally, as game designers work more closely with scientists to design games that are both fun and engaging, we can expect to see even more exciting and innovative biotic games, similar to those found in top-rated AAA games.
Biotic games offer a unique opportunity for game developers and designers. By treating cells as "living computers" and breaking down biotic games into bioware, hardware, and UI, developers can more easily conceptualize this genre and bring their creativity to life. Exciting new dimensions of play are just waiting to be discovered in this space, and I'm eager to see what developers come up with.
See you next week :)
Thanks for reading The Gameful Scientist! I’d love to hear your thoughts below.
Feel free to contact me here or chat with me on Twitter @ATrotmanGrant :)